![GettyImages-1404205939 [Converted] An illustration of a doctor’s arm and hand holding tweezers and manipulating DNA.](https://mdaquest.org/wp-content/uploads/2024/11/GettyImages-1404205939-scaled-e1731943357134-1024x512.jpg)
Gene Editing Holds Promise as Viable Therapy
By Larry Luxner | Monday, November 18, 2024
Gene therapies for neuromuscular diseases are creating quite a buzz these days. This is a promising therapeutic area, with two such therapies approved and many more in clinical trials. Some experts would argue that gene editing holds even more promise, as it can truly alter a genetic mutation at its source and avoid some of the limitations current gene therapy methods have.
At the moment, gene editing is still in the early stages. In 2023, the US Food and Drug Administration (FDA) approved the first gene editing-based therapy for the treatment of sickle cell disease. Although no therapies using gene editing are currently approved or in clinical trials for neuromuscular diseases, it’s beneficial to understand what it is and why the scientific world is excited about it.
How does gene editing work?
Gene editing involves altering a targeted section of DNA within cells. This approach can precisely target genetic mutations.
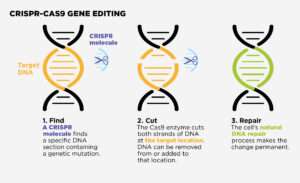
An illustration of the CRISPR-CAS9 gene editing process.
The most well-known gene editing tool is CRISPR-Cas9. This technology can enter cells, cut a specific section of DNA and then remove, add to, or replace that section. This change becomes permanent, allowing cells containing the repaired DNA to make functional proteins.
Another method being explored is base editing. This is an ultraprecise gene editing method that targets just one of the four base chemicals that make up DNA. These bases are called adenine (A), guanine (G), cytosine (C), and thymine (T). Certain bases pair with each other — A with T and C with G — to form units called base pairs. A base that is missing, mismatched, or out of order can alter how cells make proteins.
“Typical CRISPR-Cas9 editing involves cutting both strands of the DNA helix. With base editing, there’s no cut in the DNA; we simply modify a single letter in the DNA,” says Eric Olson, PhD, Founding Chair of the Department of Molecular Biology at the University of Texas Southwestern in Dallas and a pioneer in the gene editing field. “We are very excited about this approach, which we’re taking, as it does not have the concerns associated with making a double-stranded DNA break.”
MDA has funded several research studies on promising new approaches to gene editing, including base editing.
How is gene editing different from other gene therapy methods?
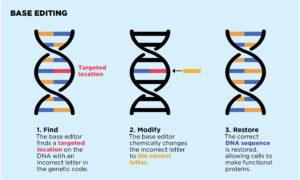
An illustration of the base editing process.
Gene editing is a type of gene therapy. Most of the currently available gene therapies work by delivering a working gene into the body using an adeno-associated virus (AAV) vector. The AAV is not harmful, but it can enter cells to deliver a working gene that provides new instructions to produce a needed protein. This is called gene replacement.
“With gene replacement, we’re trying to add a functional copy of a gene. We’re not fixing the mutation or changing anyone’s DNA,” explains Sharon Hesterlee, PhD, Chief Research Officer at MDA. “The person still has the mutated gene in their chromosome; we’re just putting in a healthy copy. The idea is that this healthy copy can stand in.”
This method of gene therapy is used in onasemnogene abeparvovec-xioi (Zolgensma®), approved by the FDA in 2019 to treat spinal muscular atrophy (SMA). This therapy has had demonstrable benefits. Some children who were identified with SMA through newborn screening and given Zolgensma as infants have not yet developed symptoms of the disease.
It’s possible these benefits will last a lifetime, but gene therapy has not been around long enough to know. Our bodies are constantly making new cells to replace old cells. With gene replacement, the new cells still contain the genetic mutation. As new cells replace older cells that received the functional copy of the gene, the effects of the gene therapy may become “diluted.” Dr. Hesterlee points out this is especially concerning with a muscle disease like Duchenne muscular dystrophy (DMD). “The child who receives gene therapy will continue to grow muscle, and that new muscle will not have the gene you delivered,” she says.
Among the biggest issues with current gene therapies using AAV vectors is that they can only be given once, even if they wear off.
“Once patients have been dosed with AAV, they can’t be dosed again — at least not currently — because of the body’s immune response,” Dr. Olson says. “There may be ways to bypass that response, but we’re not there yet.”
In addition, because AAV is a naturally occurring virus, some people have been exposed to it without knowing it and have developed immunity. They would not be eligible for any gene therapy using an AAV vector.
Advantages of gene editing
The biggest advantage of gene editing is that it truly “fixes” a genetic mutation.
“Gene editing offers the opportunity to correct a mutation within the patient’s DNA,” explains Dr. Olson, who directs UT’s Hamon Center for Regenerative Science and Medicine, as well as the Wellstone Center for Muscular Dystrophy Research. “This is particularly attractive for a disease like DMD because the dystrophin gene is one of the largest genes in the human genome,” he says. To develop an AAV-delivered gene therapy for DMD, researchers have to use a miniaturized dystrophin gene that doesn’t have the full functionality of the whole gene.
“When one uses gene editing, the corrected gene is then expressed at the right time, in the right place, and at the right level,” Dr. Olson says. “This is why we think it’s the ultimate solution for many neuromuscular diseases — but it’s going to take time and careful experimentation to ensure a safe approach.”
Challenges of gene editing
Because gene editing permanently changes DNA, it might not require redosing, but it does raise safety concerns.
“You’re introducing into the patient an enzyme that can modify the genome, though so far, we and others have not seen significant off-target editing,” Dr. Olson says.
A chance that a gene editing therapy might result in an off-target edit (an unintended genetic modification) may be considered too risky. “Even if it’s cutting in the right place 99% of the time, the problem is the 1% where it doesn’t,” Dr. Hesterlee says.
Despite these concerns, neuromuscular disease researchers continue to pursue gene editing because of the promise it offers. “If you can get it right, then you’ve done a permanent correction,” Dr. Hesterlee says.
Where gene editing research is heading
Dr. Olson’s lab is working on solving one of the major challenges of gene editing: how to deliver gene editing in vivo, or inside the body. The only FDA-approved gene editing therapy is performed ex vivo, or outside the body — cells are extracted from the person being treated, modified in a lab, and then implanted back into their body.
Currently, AAV vectors are the most effective way to deliver gene therapy in vivo, but gene editing materials are difficult to fit in an AAV. According to Dr. Olson, the long-term solution may be a combination of gene editing with nonviral delivery. This would accommodate the size of gene editing materials while lowering toxicity associated with large doses of viral vectors, which in the past has resulted in some adverse reactions in DMD gene therapy clinical trials.
Dr. Olson is encouraged by the highly successful Pfizer and Moderna COVID-19 vaccines, which rely on messenger RNA (mRNA) technology as a delivery method. Inside the body, mRNA gives instructions to cells, and it has proven to be a safe, effective, and precise way to deliver vaccines and, potentially, treatments for genetic diseases.
“We’ve certainly seen the transformative impact of nonviral approaches with COVID vaccines, though there are other challenges with muscle, which comprises 40% of the human body mass,” he says.
Dr. Olson believes he and others are close to breakthroughs on these challenges. “I’m hopeful that gene editing and other approaches will bring long-term benefits to the many patients in need,” he says.
Larry Luxner is a freelance journalist based in Israel. He writes frequently about rare diseases.
CRISPR-CAS9 Gene Editing
- Find
A CRISPR molecule finds a specific DNA section containing a genetic mutation.
- Cut
The Cas9 enzyme cuts both strands of DNA at the target location. DNA can be removed from or added to that location.
- Repair
The cell’s natural DNA repair process makes the change permanent.
Base Editing
- Find
The base editor finds a targeted location on the DNA with an incorrect letter in the genetic code.
- Modify
The base editor chemically changes the incorrect letter to the correct letter.
- Restore
The correct DNA sequence is restored, allowing cells to make functional proteins.
Words to Know
Here is a guide to terms used in this article.
CRISPR-Cas9: A tool used to “edit” pieces of a cell’s DNA. Also called CRISPR, this tool uses a specially designed RNA molecule to guide an enzyme called Cas9 to a specific sequence of DNA. Cas9 then cuts the strands of DNA and removes a small piece, causing a gap where a new piece of DNA can be added.
DNA: A long molecule that carries a cell’s genetic information.
Genes: Segments of DNA that act as blueprints for making proteins for virtually every structure and function in the body.
Gene editing: A type of gene therapy in which a particular segment of DNA is removed or altered.
Gene therapy: Adding, removing, or changing genetic materials in a person’s genetic code to treat or cure a disease.
Genetic mutation: A flaw in a sequence of DNA that alters gene function.
Genome: The sum of all genes in the body.
Messenger RNA (mRNA): A molecule that contains instructions for cells to make proteins.
Proteins: Complex molecules that play many critical roles in the structure, function, and regulation of the body’s tissues and organs.
Vector: A delivery vehicle that carries new genes to cells
Next Steps and Useful Resources
- Stay up to date on Quest content! Subscribe to Quest Magazine and Newsletter.
Disclaimer: No content on this site should ever be used as a substitute for direct medical advice from your doctor or other qualified clinician.