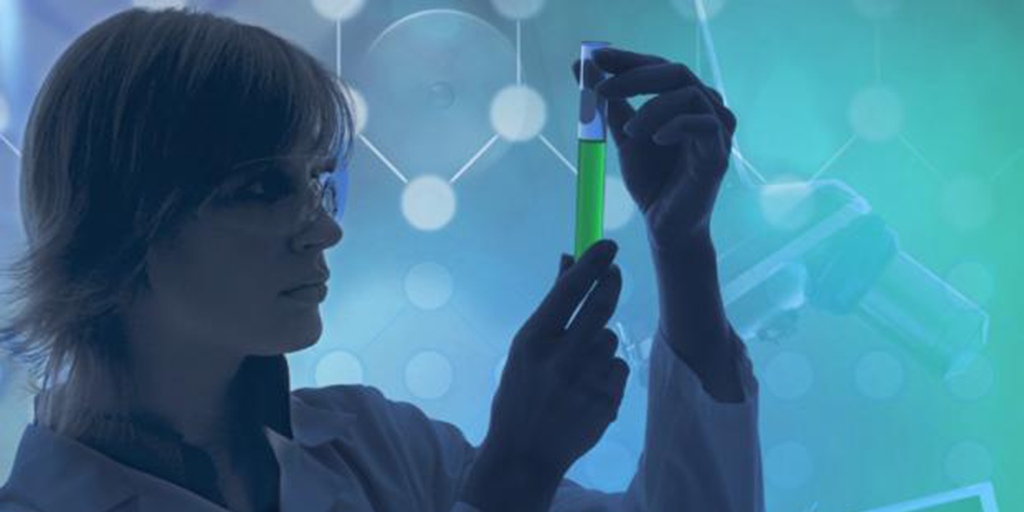
ALS: Not Just About Motor Neurons Anymore
By Margaret Wahl | Friday, April 30, 2010
Motor neurons are lost in ALS, but new evidence shows they don’t die without significant help from their neighboring cells. These neighbors may be easier to target with therapies than the motor neurons themselves.
Not long ago, if you asked any neurologist about ALS, you’d have been told that it’s a disease of the motor neurons — nerve cells in the brain and spinal cord that control voluntary muscle movement. That’s still true. But the last few years have added clauses to that description that recognize the important contributions of other cells to the deadly disease process. That could be good news, because those other cells may be easier targets than motor neurons.
‘Protect me’ versus ‘eat me’ signals
Stan Appel, a longtime MDA research grantee who directs the MDA/ALS Center at Methodist Neurological Institute in Houston, is convinced by recent evidence that immune system cells require immediate attention in understanding and treating ALS.
Granted, a lot of that evidence comes from a mouse model of ALS called the SOD1 mutant mouse. The mouse has a mutation in the SOD1 gene, a problem that causes ALS in only 1 percent to 3 percent of human patients, but Appel thinks the SOD1 mouse model has much broader implications for human ALS than those numbers imply.
He thinks the problem in both familial ALS (which is caused by mutant SOD1 genes) and in sporadic ALS (which doesn’t have a clear cause) is misfolded proteins — either SOD1 itself or other misfolded proteins that cause trouble. He calls these “rogue” proteins, and he thinks they activate the part of the immune system that’s overseen by microglia, which carry out immune surveillance inside the central nervous system (the brain and spinal cord).
Appel cites a recent set of experiments showing that, in SOD1 mice, mutant SOD1 is not directly toxic to motor neurons but requires activation of microglial cells in their surroundings. Motor neurons, at least in the SOD1 mouse, do not die alone, but with the help of microglia.
Appel thinks that’s what happens in sporadic ALS in humans as well. “If an injury signal comes from a motor neuron, it activates the microglia in a negative way and can kill motor neurons.”
Appel cites recent findings by the MDA-supported ALS Therapy Development Institute (ALS TDI) which show that a part of the immune system is overactive in human ALS blood samples, and some evidence from human ALS tissue samples that misfolded proteins may be a source of the problem.
“There are antibodies [proteins that can act like magnets] that can be used to recognize misfolded, oxidized [chemically modified] SOD1,” Appel says, citing the work of several researchers. “And if you look at a slice of tissue from a patient with sporadic ALS, these same antibodies seem to recognize misfolded, oxidized proteins, possibly even oxidized SOD1.”
But microglia don’t act alone either. They take some of their marching orders from T cells, immune system cells that travel throughout the body via the bloodstream and can either augment or dampen an immune response.
Recently, Appel’s group did a set of experiments in SOD1 mice in which they got rid of all of the functioning T cells in the mice. Some people expected the mice to do better without T cells, figuring that T cells were toxic and that in their absence the mice would live longer. But the opposite happened. The mice had a rapidly progressive ALS-like disease and died earlier than animals with T cells.
That, says Appel, told the researchers that more of the T cells were protective than harmful. He says evidence shows that early in the course of ALS, T cells and the microglia tend to protect damaged motor neurons, but later in the disease, they turn against the motor neurons.
“We don’t know exactly when the switch occurs,” Appel says. “But after a time, when all this injury to motor neurons from rogue proteins is taking its toll, the motor neuron says to the microglial cells, ‘You’ve done your best to protect me, but now you just better take me out quietly.’ The signal changes from a ‘protect me’ signal to an ‘eat me’ signal.”
Experiments with immunosuppressive drugs in ALS go as far back as the 1980s, when Appel’s group tested cyclosporine in people with ALS. It wasn’t helpful, and Appel says he thinks he knows why.
Cyclosporine and many other immunosuppressants don’t directly affect microglia, he says. They affect T cells — all of them, including those that protect and those that injure motor neurons — and removing protective T cells is not a good thing in ALS.
“If we suppress all the T cells, we’re suppressing the good guys and the bad guys,” Appel says. “If in fact the good T cells come in and modulate the microglia and convert them into good microglia that are going to be protective, and if they modulate other T cells so that they’re good guys, and if they protect the motor neuron, then we’re doing great. But if the bad guys come in and they activate microglia to kill motor neurons, and they cause other T cells to do that, then you can see how things will go.”
What’s desperately needed, he says, is a more targeted approach — not immunosuppression, but immunomodulation, which is what the ALS TDI researchers hope their new compound will provide.
Meanwhile, Appel’s team is barking up another branch of the immune system tree. “We’re doing something different,” Appel says of his own lab’s work. “We’re talking about trying to develop good guys rather than block the bad guys.”
Astrocytes: Underperformers that also may be killers
For Jeffrey Rothstein, MDA research grantee and director of the MDA/ALS Center at Johns Hopkins University in Baltimore, the focus is on another cell — the astrocytes, also known as astroglia or astroglial cells. (The term “glia,” the Greek word for “glue,” reflects the fact that these cells and microglia were once believed to provide mainly gluelike support for nerve cells.)
Since the early 1990s, Rothstein has been looking at astrocytes in relation to ALS. His group’s focus has been on astrocytes’ role in recycling and regulating levels of the nervous system chemical glutamate, which is necessary for transmitting signals but is toxic in excess.
Rothstein and others have conducted numerous studies over the past 15 years or so, in rats and mice with an ALS-like disease and in samples of brain and spinal cord from humans with ALS. These studies show glutamate transport (the process by which the chemical is cleared away) often is deficient in this disease.
But, says Rothstein, newer evidence shows that glutamate regulation isn’t the whole story when it comes to the support that astrocytes give to motor neurons.
It seems that under normal circumstances, astrocytes also supply a nutrient called lactate to motor neurons. In ALS, they may be underperforming in this role, in addition to not taking up glutamate the way they’re supposed to.
“Astrocytes are not providing motor neurons with enough lactate, as best we can tell,” Rothstein says. “That’s defective in sporadic ALS patients, as well as in our mouse models. If you take away the ability of an astrocyte to give a neuron lactate, the neuron starts dying. So this is, unexpectedly, another pathway by which astrocytes support neurons.”
Rothstein points out that other investigators have recently found that astrocytes are not only delinquent in their duties with respect to sweeping up glutamate and supplying lactate, but that they also may be secreting something that’s directly killing motor neurons.
“The challenge is, what are they secreting?” Rothstein says. “What’s making the neuron become sick? That’s not known yet, but there are efforts under way.”
Rothstein says there are at least two ways to go about dealing with misbehaving or underperforming astrocytes: One way is to replace them with new astrocytes, and the other is to understand the various pathways by which they become dysfunctional and then fix them.
The first approach could involve transplanting new cells, such as stem cells that give rise to astrocytes. This approach has its proponents, but also its detractors.
If astrocytes are messing things up, particularly if they’re mainly being delinquent, Rothstein says, “It does make sense to say, ‘let’s just put in new astrocytes, pure astrocytes.’ That’s the stem cell approach.”
But, he says, “We’ve identified problems with that. How do you inject the cells? How do you deliver them to the entire nervous system? That’s a huge limitation.”
But there’s another way to get new astrocytes that doesn’t involve cell transplantation. “Your nervous system has adult stem cells both for neurons and for glial cells,” Rothstein says, noting that the latter type of stem cells haven’t gotten as much attention as he thinks they should.
“These cells, called NG2 cells, are enormously numerous in humans and in animals,” he says. “If you look at a stain for these cells, you’d think you’re looking at astrocytes. But they’re not astrocytes. Even now, no one knows what they normally do. But one thing we do know now is that, during development, they can turn into new astrocytes. Our hypothesis is: If they normally can do this, at least at one point in time, what if we could take drugs and use them as the inducing switch to turn them into new astrocytes?”
That approach, which Rothstein has MDA funding to pursue, would accomplish the same thing as transplanting new astrocytes into the nervous system, he says, but without having to inject cells into the nervous system.
“You’d be treating someone with a drug that briefly activates these cells and tells them to turn into astrocytes, so you essentially repopulate part of the nervous system with fresh, baby astrocytes. It’s a drug-based therapy, but it’s a stem cell therapy.”
Meanwhile, Rothstein says, it’s still worthwhile to try compensating for the things that astrocytes aren’t doing or are doing wrong in ALS.
The drug ceftriaxone, now being tested in people with the disease, is believed to increase production of a glutamate transporter by astrocytes. Rothstein says he was part of the finding about increased glutamate transport from ceftriaxone, but now, he says, “that’s actually not the only thing it does. It turns on lots of genes. Could some of those actions be antiglutamate? Yes. But it could be other things, such as dampening down inflammation.”
Being able to track what’s going on in and around astrocytes in human patients by using positron emission tomography (PET) scans would provide a great advantage for scientists, Rothstein says.
So his group is looking at developing radioactive tags (“ligands”) for specific astrocyte proteins, such as glutamate transporters, that would light up in a PET scan.
“Lots of companies are using PET ligands to do what’s called ‘pharmacodynamics,’ the study of the effects of a drug on the body,” Rothstein says. “They want to know: Does the patient have a problem in this pathway, and if so, is the drug working?”
For example, he says, only some, not all, ALS patients have a loss of glutamate transporters. “So,” he says, “first and foremost, let’s find the ones that do, and then secondly, let’s find which patients respond to our drug that increases transporters. Having this PET ligand would tell us that.”
Disclaimer: No content on this site should ever be used as a substitute for direct medical advice from your doctor or other qualified clinician.